January 25,2016
On this day we conducted an experiment on reflection and refraction.
The conducted experiment had three objectives. Using an optical disk, we were to investigate the reflection and refraction of light. Another objective was the measurement of the index of refraction of a material using the optics set-up. Lastly, we were to trace the path of light as it emerges from optical materials of different geometries.
We were supposed to do an experiment on thermodynamics instead of this experiment.
I love light. It amuses me. I was excited to do the experiment because it dealt with the properties of light.
Before we started doing the experiment, we first borrowed the materials that were to be used.
The first part of the experiment was the alignment of the optics. Basically, the light source and the optical disk were mounted on the optical bench with the slit plate and slit mask in between them, respectively. A single ray coincident on the zero degrees-zero degrees axis of the optical disk was to be produced with the set-up orientation. This part was not very hard at all.
The second part of the experiment was to do the reflection by plane and spherical mirrors. The plane mirror was placed on the disk such that the mirror coincides with the 90 degrees-90 degrees axis of the optical disk. It was made sure that the center of the mirror and the center of the optical disk coincided. The optical disk was rotated such that the light would hit the plane mirror at 0,15 and 30 degrees. The angles at which the light was reflected were recorded. The same procedure was done for the convex and concave mirrors. Table 1 below shows the obtained angles. It can be seen that the angle of reflection is the same as the angle of incidence when light is to be focused on a mirror.
Table 1. Refraction by plane and spherical mirrors
The second part of the experiment dealt mainly with the refraction in glass. The mirror was replaced with a cylindrical lens. The flat surface of the glass coincided with the component axis of the optical disk. The alignment of the centers was made sure. The optical disk was rotated 5 times such that the light would hit the semicircular glass at 10,20,30,40 and 50 degrees. The index of refraction of the glass was calculated using Snell’s law.
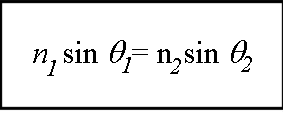
Figure 1. Snell’s Law. Picture obtained from : 12physics.blogspot.com
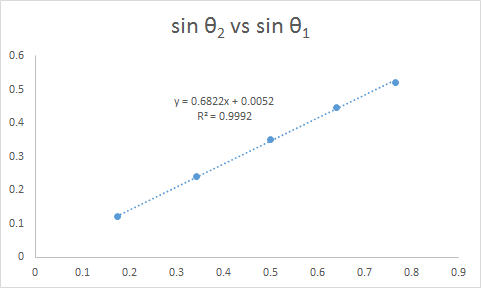
Figure 2. Relationship of the sines of the angles of incidence and refraction when incident ray strikes flat surface
By looking at the trend line in Figure 2, it can be seen that the slope is 0.6822. This means that the ratio of the indices of refraction of gas and the glass is 0.6822. Further calculations using Snell’s law would give us the index of refraction of the glass which is 1.47.
The next part of the experiment was to rotate the optical disk ( not the semicircular glass) by 180 degrees such that the incident ray strikes the curved side of the glass. It was made sure that the incident ray would strike the curved surface and pass through the center of the disk and the center of the flat surface for the reflected and refracted ray to coincide with the 0 degrees-0 degrees axis. The difference of this set-up with the previous one is that which medium comes first. In this set-up, the incident ray strikes the curved surface. In the previous set-up, the incident ray strikes the flat surface.
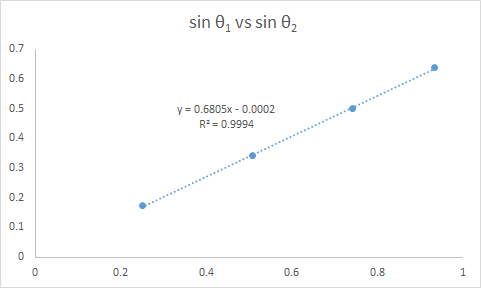
Figure 3. Relationship of the sines of the angles of incidence and refraction when incident ray strikes curved surface.
Based on Figure 3 and with the corresponding calculations using Snell’s law, the obtained index of refraction is still 1.47. This means that regardless whether the curved surface is struck first or not, the index of refraction would not change because it is a property of the glass. What would change is the angle of refraction.
The last part dealt with total internal reflection. The set-up from the previous part was still used. The optical disk was rotated and the angle at which the refracted ray is parallel to the flat surface was to be found. It can be seen in Table 2 that the critical angle was found to be 43.5 degrees. The index refraction the glass can be obtained by plugging in the critical angle as θ1 and 90 degrees as θ2. The speed of light inside the semicircular glass was calculated using definition of the index of refraction : the ratio of the speed of light in vacuum and speed of light in a medium.
Table 2. Total internal reflection
Overall, the experiment was fun and easy. I hope the experiments to come will be easy and fun as well.
Woo! Achievement unlocked! First blog ever! First blog post ever!